From Earth is a Nuclear Planet by Mike Conley (Author), Tim Maloney Ph. D. (Author), Stephen A. Boyd Ph. D. (Scientific Editor)
Author: THE KERNEL
Listen to the audiobook version here!
You’ve probably heard of radioactive half-lives, which can be anywhere from a nanosecond to several billion years. The half-life of a radioactive material has elapsed when one half of any given batch of the stuff changes into a different isotope, or a completely different element.
Some of the hazardous materials in science and industry are toxic forever, like the cadmium in coal ash, which is also found in solar panels. One good thing about radioactive material is that it’s in a constant state of decay—a continual process of becoming benign.
The original material decays by changing into one radioactive isotope after another, taking any number of zigzag paths down the Periodic Table (Fig. 18) until it finally becomes a stable, non-radioactive element. Uranium and plutonium, for example, usually become lead (“Pb” in the decay chain below, from the Latin word plumbum, from which we get the word plumbing).
Of course, the larger the original batch, the more total radioactivity there is. But regardless of the size of the batch, at the end of one half-life, half the radioactivity will be gone. As a general rule of thumb, ten half-lives will render a batch benign: The radioactive half that remains after the first period will be reduced by half in the second period, and so on, until the original batch becomes a lump of radioactively benign stuff and virtually all the zoomies are gone.
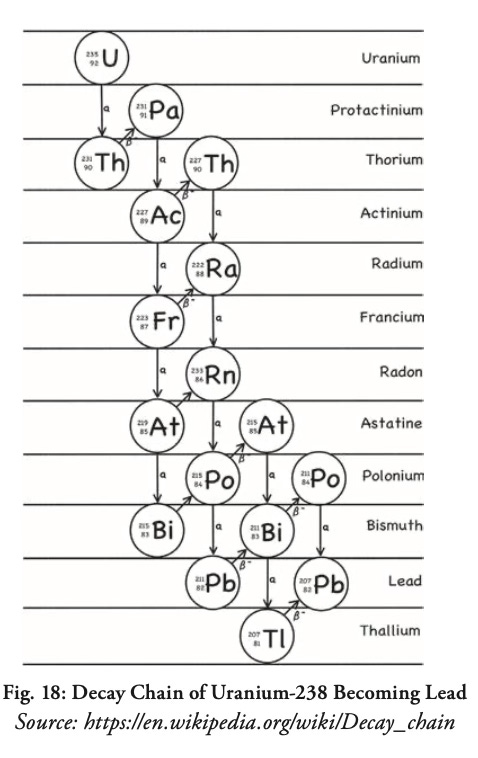
Check out the graph below. After four half-lives, the material is only 6.25% as radioactive as it was when starting out. Total radioactivity will of course depend on the size of the original batch, but if you extrapolate the math, ten half-lives result in less than 0.1% (one one-thousandth) of the original amount of radiation.
For example, the half-life of plutonium-239 is 24,000 years. This means it will be considered inert after it ages for about 240,000 years, long enough for virtually the entire batch to transmute into stable, non-radioactive lead. Of course, a better solution than burying it for a quarter-million years might be to use it as fuel in a fast-neutron reac- tor. This will break it down into much shorter-lived fission products (FPs), and we’ll get loads of clean energy for our good deed.
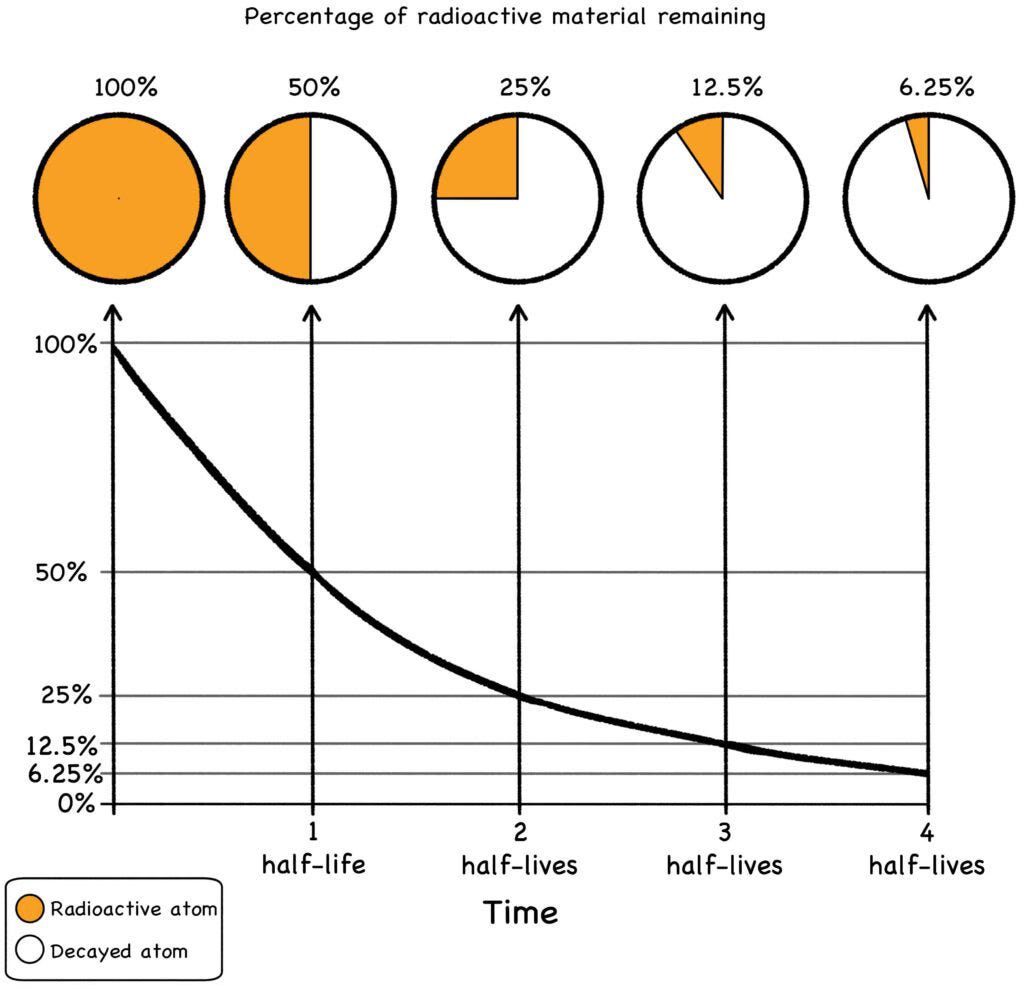
Fig. 19: Half-lives
Source: SW Infographics / Knowable
3.1 RECYCLING FOR FUN AND PROFIT
If you’re a fan of the environment, you’ll be happy to know that recycling used reactor fuel would do far more to reduce society’s waste stream than our ongoing efforts to recycle plastic. Petroleum industry hype to the contrary, our efforts over the last thirty years to recycle their plastic waste have largely been greenwashing.
In 1989, the Exxon Valdez spilled 257,000 barrels of crude oil into Alaska’s Prince William Sound. [1] The disaster caused more environmental damage than any spill in history, including the larger-volume Deepwater Horizon blowout of 2010. The Valdez incident sparked global outrage and a determined pushback against petroleum and petrochemicals, including plastic.
After Chernobyl in 1986 and the Exxon Valdez in 1989, interest in renewable energy understandably soared. Sensing a long-term sea change in public opinion, Big Oil began promoting municipal recycling programs. [2] They figured if people could start going green by cleaning up plastic waste, maybe we would all be hating on petroleum a little bit less, because the plastic made from it is kinda / sorta recyclable. Many of us bought into the idea, chauffeuring the kids to school in gas-guzzling SUVs and diligently recycling the trash at home.
Thankfully, there is something that would actually help: By using the energy stored in used reactor fuel, municipalities could power the carbon-free recycling of all their waste, including plastic. A plasma-arc smokeless furnace (Fig. 20) operating at 1,800o C will cleanly reduce any waste down to its constituent metals, mixed vitrified solids (glass), ethanol and methanol, both of which are useful as carbon-neutral fuels. This is not incineration; this is the molecular-level breakdown of any and all municipal trash—medical waste, hazardous material, food waste, even Mom’s old couch—without smoke, chemical residue, or other pollution. [3]
The drawback of plasma-furnace waste remediation has always been the enormous amount of electricity required. A dedicated fleet of fast-neutron reactors, running on “spent” nuclear fuel (SNF), could resolve this dilemma, powering a 21st-century solution to a 20th-century problem. The strategy is elegantly simple:
Use nuclear waste
to power the cleanup
of all our other waste.
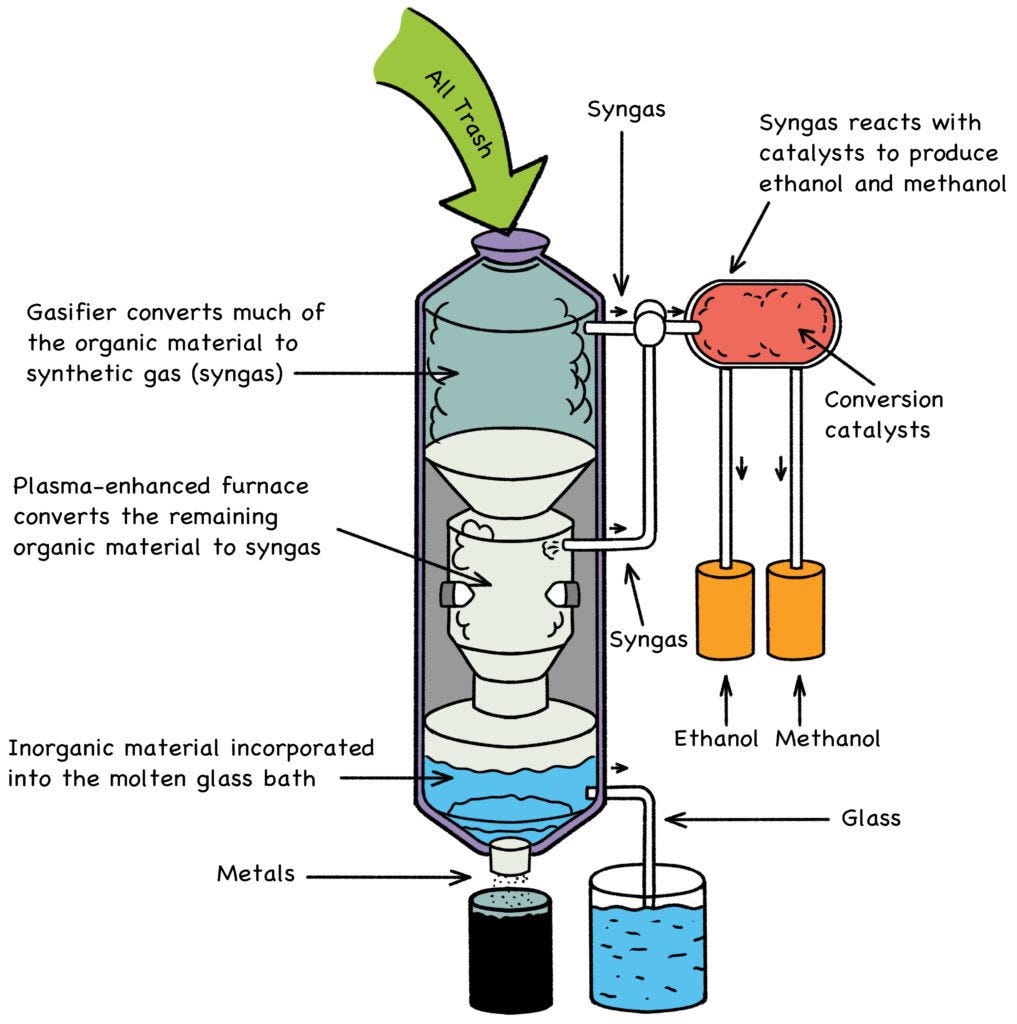
Fig. 20: Plasma-Arc Smokeless Trash Incinerator
Source: https://energy.mit.edu/news/turning-waste-into-clean-fuels/
3.2 TURNING BIG ONES INTO LITTLE ONES
Fissioning (splitting) a big fuel atom to release the energy locked in its nucleus results in two (sometimes three) smaller atoms. Though these fission products (FPs) are unstable and therefore radioactive, they have much shorter half-lives than the fuel atom from whence they came. As the philosopher Lao Tzu famously observed: “The flame that burns twice as bright burns half as long.”
Some of these short-lived fission products can shed copious amounts of thermal energy. This is a good thing, because they can be chemically extracted from spent fuel and used to produce thermoelectric energy—the direct conversion of heat to electricity. For example, Russia has been using slugs of strontium-90 reactor “waste” to electrify their remote lighthouses. The amount required is small enough to fit in a tall Red Bull can. Think of it: Enough energy to power a lighthouse for decades, in the palm of your hand. So where’s the waste? [4]
Used reactor fuel can also be harvested for the isotopes used in medicine, science, and industry. Examples include technetium-99m for monitoring blood flow, chromium-51 for blood cell tagging, cobalt-60 for sterilizing harmful bacteria, and strontium-90 for precision thickness gauges.
When it comes to used fuel, or any kind of nuclear “waste,” always keep one thing firmly in mind:
It’s only waste if we waste it.
Plutonium-238, made from the Neptunium-237 extracted from used reactor fuel, powers the Mars rovers and all deep-space probes. Voyager II, for example, was launched nearly a half century ago and has finally left the solar system. After 44 years, the probe is still operating at 70% power, and has recently been reprogrammed to explore interstellar space. [5] You can’t boldly go with solar panels.
Since a radioactive atom, by definition, actively radiates (ejects) pieces of itself, it follows that any radioactive source can only spit out a finite number of zoomies before it stops being radioactive. It can’t just keep creating new zoomies out of thin air. Which means that the longer the isotope’s half-life, the less radioactive it is.
That may seem counterintuitive at first, but upon reflection it soon becomes clear. And yet, half-lives are one of the most widely misunderstood aspects of nuclear science, and are routinely used to scare uninformed people: “OMG! Thorium is radioactive for fourteen billion years!” (Zoomies! We’re doomed!)
Take a breath, good citizen. A half-life of fourteen billion years means that thorium is so weakly radioactive, it will take that long for one half of any given batch of the stuff to eject the occasional zoomie as it ever so sloooooowly turns into lead. The same billion-year scenario applies to potassium and natural uranium.
3.3 LEAVE NO FUEL UNBURNED
EROI (pronounced E-roy) means Energy Returned On Investment. The term refers to the total amount of energy invested in building, fueling, operating, and decommissioning an energy production device, relative to the total amount of useful energy derived from said device over its lifecycle. [6]
You’ll also see it spelled EROEI, Energy Returned On Energy Invested, which some feel is a better phrasing, but the original EROI acronym stuck. Charles Hall, the noted ecologist who coined the term, explains EROI with this simple formula:
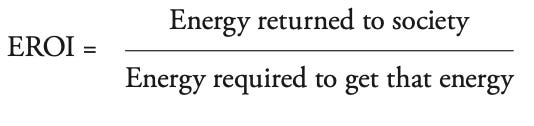
As shown in the graph, the EROI for a fast-neutron reactor (see Chapter 5) can be a whopping 2,000 to one. ThThe skinny detail on the right shows the actual scale—the energy density of a fast reactor is literally offff the chart. In stark contrast, the EROIs of solar, wind, and biomass are in the single digits.
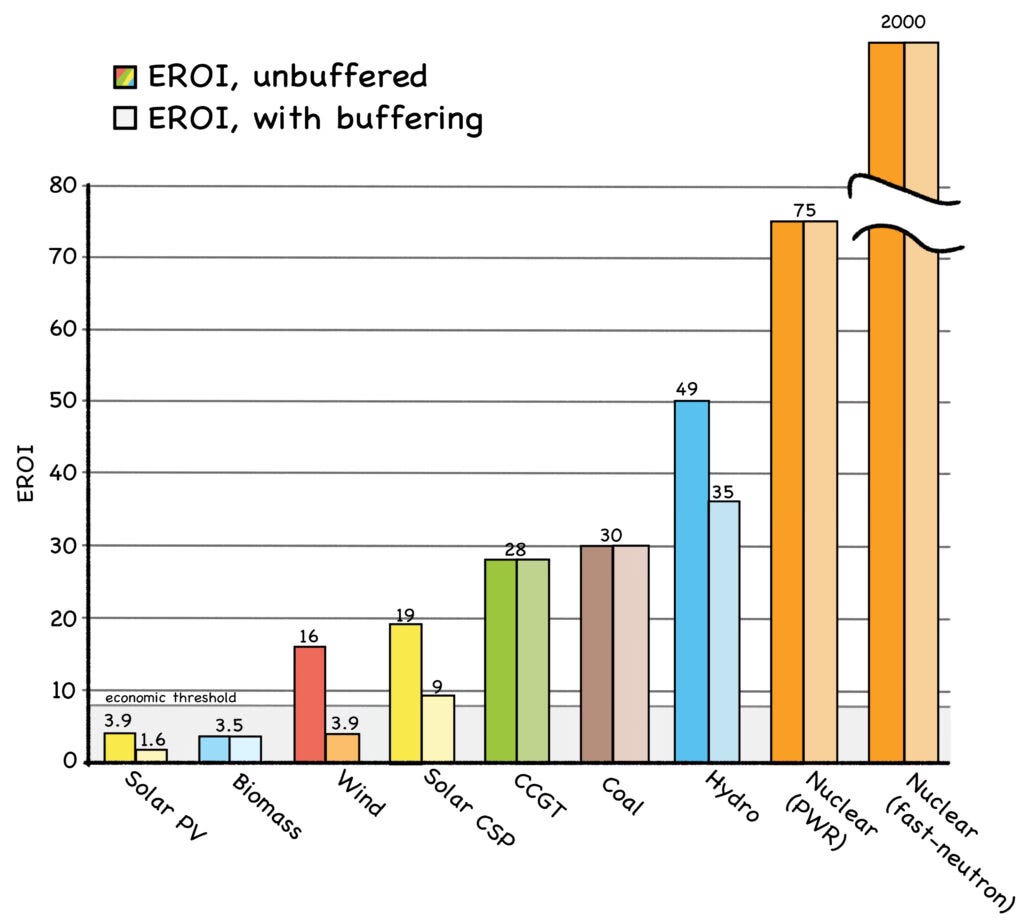
Fig. 21: EROI Energy Returned on Investment
Source: https://festkoerper-kernphysik.de/Weissbach_EROI_preprint.pdf
Notice that the EROI numbers for renewables are even worse when “buffering” is factored in. This term refers to the backup provided by energy storage or other power plants. The even-lower EROI values for buffered renewables are due to the energy it takes to build and maintain the buffering device.
NERD NOTE: The buffering of intermittent energy (by storing it in a battery, for example) is like the buffering in a video player, and for much the same reason: Stuff arrives in bits and pieces and is allowed to build up in storage, so it can then be smoothly streamed without interruption.
EROI analysis has been criticized because as technology improves and prices drop, EROIs improve as well. Instead of 19 for wind and 3.9 for solar, as shown in the 2014 graph above, their estimated EROIs in 2020 ranged as high as 25:1 (Fig. 21-A). But this misses the larger point: As wind and solar become more than 20% of our energy mix, they’ll need more buffering than our conventional power plants now provide for free. And as they supply more and more of their own buffering, their EROIs will drop off a cliff.
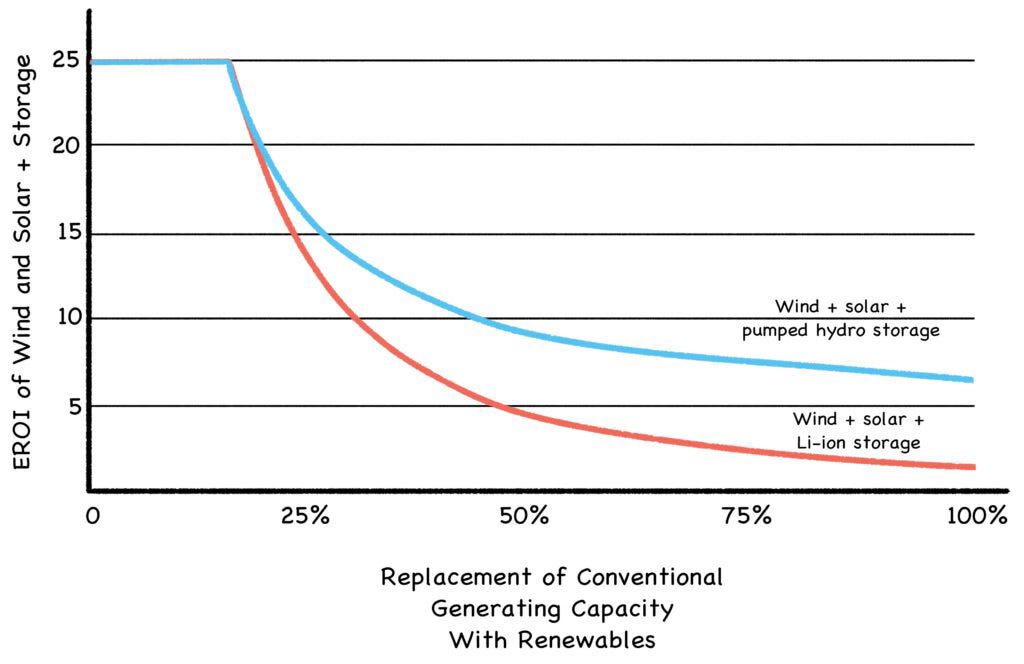
Fig. 21-A: The EROI Cliff
Source: Energy Storage and Civilization (Palmer and Floyd, 2020) https://link.springer.com/book/10.1007/978-3-030-33093-4
Adequate buffering for renewable systems is a substantial expense, but is often dismissed as an externalized cost. Indeed, renewables can seem quite affordable until the buffering required to stabilize their erratic performance (grid-scale batteries, gas power plants, etc.) is added to the sticker price. Most fans of renewable energy haven’t quite absorbed this sticker shock, and what it portends for the future. (We explore the details in Roadmap to Nowhere.)
The EROI threshold for a viable power source in a developed society is an energy investment ratio of at least 7:1. [7] This means that over its service life, an energy source must produce at least seven times the energy that was invested in building, operating, and decommissioning the thing. If it can’t do that, then it’s not fit to serve as a utility power plant in a modern economy (more on this in Chapter 7).
Charles Hall points out that with an EROI of 3:1 or even 5:1, you couldn’t do much more than power a civilization like England had in the 16th century. [8] At that time, up to 50% of the economy revolved around fuel. While some wood was available for space heating, most of it was prioritized for forging iron tools and weapons. Civilization grew at a snail’s pace, with little energy available for anything more than basic subsistence.
The introduction of coal in the 1750s made the steam engine practical. With the wide availability of coke (refined charcoal, purified by heating coal without burning it), the high temperatures for steel production became feasible. In the market economy that coal and steam created, fuel’s share fell to about 25%.
With the expansion of petroleum around the turn of the 20th century, fuel’s portion of the modern market economy gradually shrank to around 5%–10%, even as the world became electrified and mobilized. That’s what energy density is all about.
For a developing society, or a low-industrial society, an EROI below 7:1 may suffice. But if a society intends to advance beyond basic necessities, they must grow their capacity for energy production as a crucial first step. Energy is what drives economic progress and the advance of civilization. The steam engine came before the Industrial Revolution, not the other way around. Energy comes first.
Learn More About Earth is a Nuclear Planet Here
Pre-Order the Book Today!