The Nuclear Heartbeat of Space Exploration
As soon as we leave Earth’s orbit to wander deeper into space, nuclear power is our best and often only choice. Here is why.
Written by Christina Unger
April 22, 2020
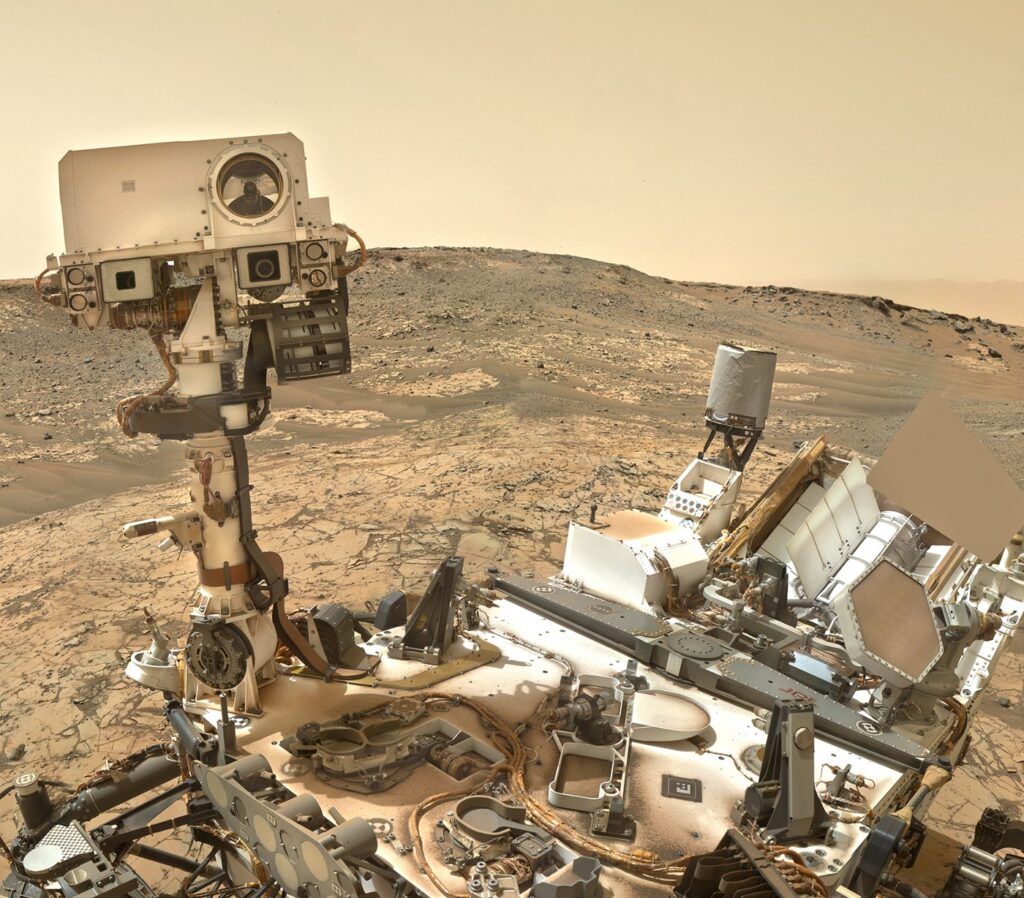
When Voyager left our solar system and reached interstellar space in 2012, it had traveled billions of kilometers for over 40 years and counting, on a journey that is not over yet. Voyager still calls home and is expected to do so until 2025.
The power source that keeps Voyager’s computers, scientific instruments, communication equipment and heating going is the same kind of power source that has sustained the Curiosity rover since its touchdown on Mars, and that enabled New Horizons to reach Pluto. In fact, it’s the same kind of power source that runs many of our space probes and rovers: nuclear batteries.
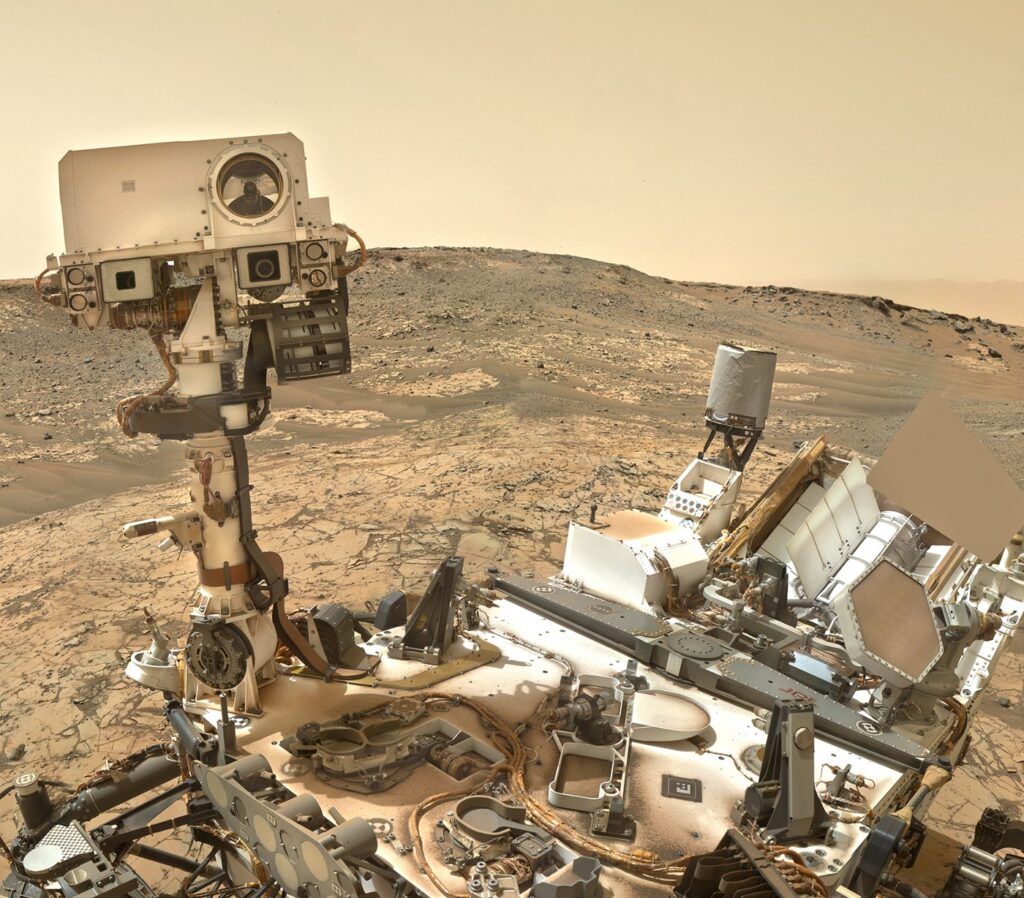
“Can’t stop. Won’t stop. A rover’s gonna rove, rove, rove, rove, rove…” Curiosity, happily taking selfies on Mars since 2012. The white, finned “tail” is its nuclear battery. (Image: NASA/JPL).
It might be surprising, but for the long-term operation of onboard systems, there are currently only two viable power sources: solar power and nuclear power.
And when conditions get really harsh, nuclear power is the only option left.
Nuclear power like in a nuclear power plant?
Not quite. Nuclear reactors start a controlled chain reaction by bombarding uranium atoms with neutrons. When a uranium atom is hit by a neutron, it splits and releases heat and other neutrons. These neutrons split further uranium atoms, releasing more heat and neutrons, and so on. This process is called fission, and the heat released is used to generate electricity.
But even when left alone, the atoms that make up uranium or other radioactive materials will split. This is a natural process known as radioactive decay. It occurs much slower than under neutron bombardment, but it still releases enough heat to create a small amount of power — the power of a nuclear battery, and enough to run space vehicles for a long time.
Imagine you’re out camping. A nuclear reactor is like the campfire: it kicks out some serious heat, enough to keep you warm, dry your clothes, boil a big pot of water and roast food. You can keep it going as long as you like by feeding it more fuel. A nuclear battery, on the other hand, is like the hot embers that get you through the night: enough heat for toasting some marshmallows, but nothing too energy-hungry. And when the embers burn out, that’s it.
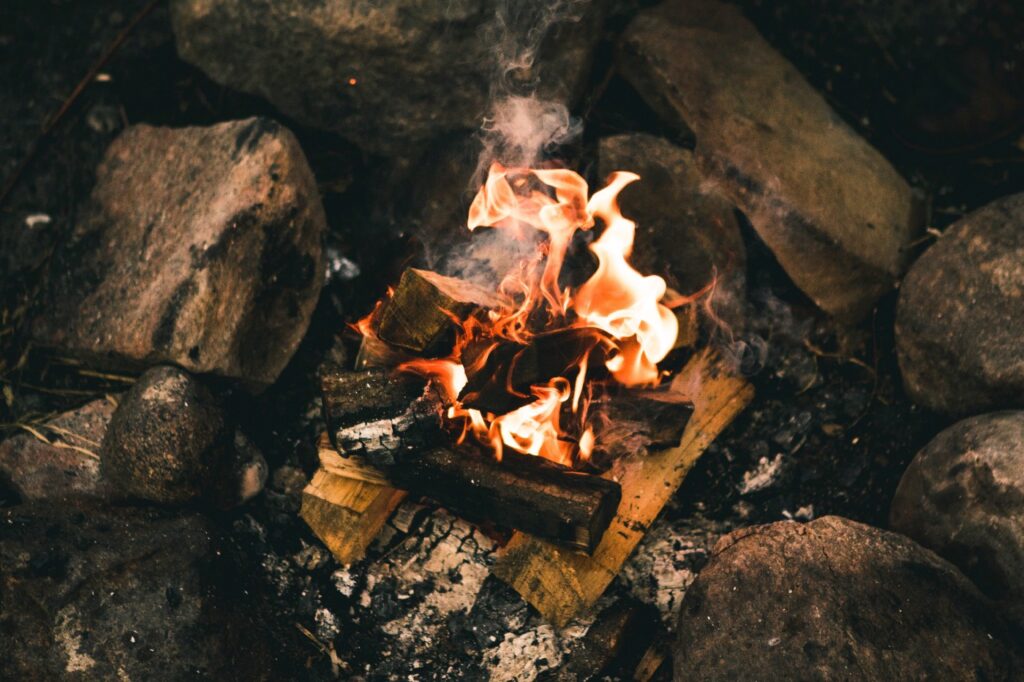
The best materials for making a nuclear battery are created inside a nuclear reactor, so a nuclear battery really is like the hot embers of a nuclear reactor. (Image: REVOLT on Unsplash)
The technical name of a nuclear battery is radioisotope thermoelectric generator, or RTG for short. Its core is an assembly of pellets containing some radioisotope, usually plutonium-238. (In case you’re wondering: This is not the kind of plutonium used in nuclear bombs.) As the plutonium atoms naturally split they give off heat. This heat is converted into electricity using thermocouples, which are wrapped around the plutonium assembly.
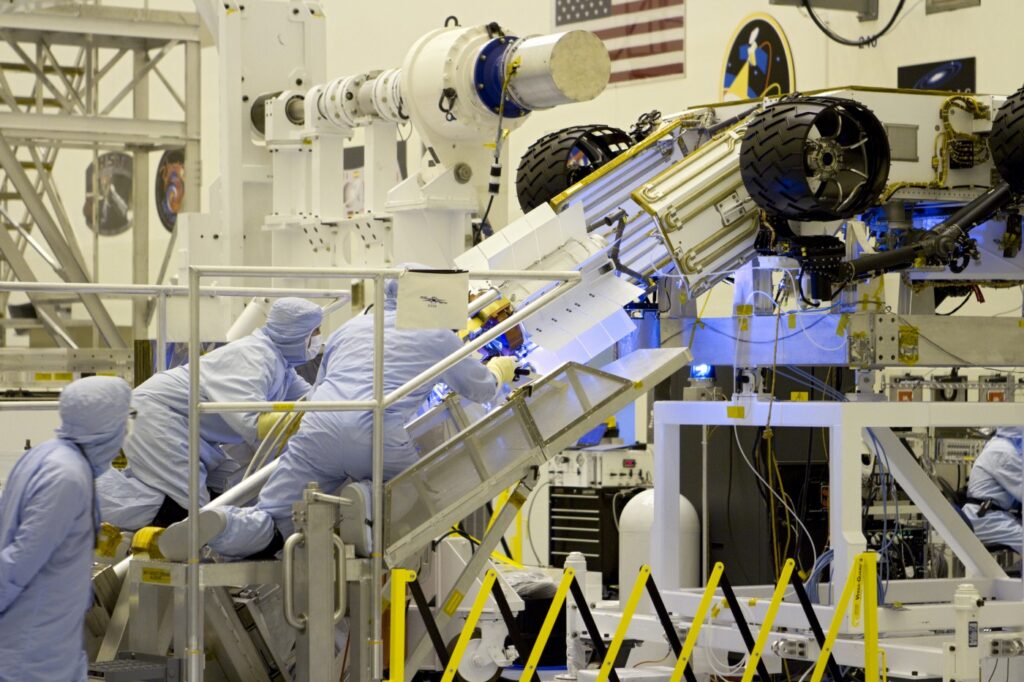
NASA technicians making sure that Curiosity’s RTG fits as expected. (Image: NASA, Wikimedia Commons)
RTGs evolved over time, as missions needed more power and regulations demanded stronger safety features. The plutonium assemblies got bigger and more modular, materials for thermocouples more efficient, and the plutonium pellets were encased in multiple layers of protective materials to shield against radiation and prevent release of materials in case of damage. The underlying principle, however, remained unchanged.
The good
There are three main requirements a power source needs to satisfy in deep space, and nuclear batteries are the only one to ace all three.
Independence from sunlight
While solar energy is the undisputed first choice in Earth orbit (the only exception being Soviet military satellites), there are lot of places in the solar system where sunlight is simply not strong enough. Jupiter is the farthest we have traveled using solar arrays. By the time you reach Saturn, sunlight is only 1% as strong as it is on Earth. Even close to home, conditions are not always favorable: Lunar nights, for example, last about two weeks.
Things would look brighter on Mars, you might think. Mars gets about one third the sunlight that Earth gets, but winter days are short and gloomy. On particularly bad days, the solar-powered rovers Spirit and Opportunity had reduced power levels that granted them only a few minutes of operation.
Mars also has dust storms that coat solar panels with layers of dirt. Both rovers had record-breaking lives on Mars, but eventually fell prey to the Martian sand. Spirit had to be given up after it got stuck and was unable to collect enough sunlight to charge. Opportunity went silent after a violent planetary dust storm. From there on, NASA rovers would be powered by nuclear batteries, not solar panels.
ESA learned the same lesson an even harder way when its probe Philae made a few mistakes during its historic landing on a comet, ending up in a shady spot where it was unable to collect enough sunlight. It lasted 60 hours, then the onboard batteries were depleted. It took seven more months before Philae had gathered enough energy to briefly communicate again.
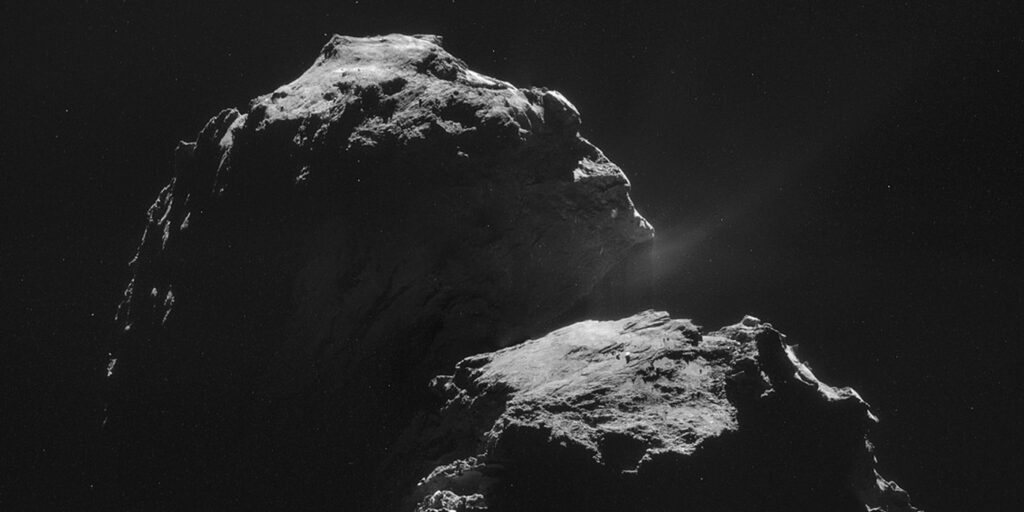
The comet 67P/Churyumov-Gerasimenko, on which Philae landed. With RTGs onboard, light or shade would not have mattered. (Image: ESA)
Nuclear batteries aren’t troubled by insufficient sunlight. Nor are they phased by the low temperatures of deep space or distant planets. (And it can get very cold in space.) In fact, the heat produced by radioactive decay is not only used to generate electricity but also to heat the electronics of a spacecraft or rover, thus preventing them from freezing.
Reliability
When a spacecraft leaves Earth, it also leaves behind any option of maintenance and repair. This means that all its components must function reliably for years without a human hand touching them. Because nuclear batteries are basically a block of plutonium wrapped in some material, there are no moving parts that can wear and there is really not much to maintain.
Nuclear batteries are used in some places on Earth for the very same reason: to provide power in remote areas where both sunlight and human presence is sparse
Energy density
Energy density is a measure of how much energy you can get for a given mass of fuel. This matters especially for space exploration, because it costs a lot to launch something into Earth orbit and beyond. When every gram counts, you want to get as much power as possible out of the fuel you take.
Nuclear is by far the most dense power source we have. It beats chemical reactions, such as burning gasoline or coal, by an order of millions.
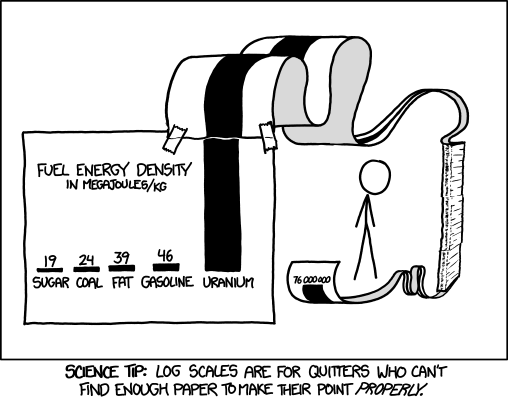
(Image: xkcd.com)
Curiosity’s 3 kg (6.6 lbs) of plutonium has delivered energy for close to eight years now, and is designed to last for at least six more. The plutonium also provides the heat that keeps Curiosity warm, meaning no extra electrical power is needed for heating.
The bad
Nuclear batteries contain radioactive material and therefore come with the risk of contamination in case of leaks, launch accidents, or uncontrolled re-entries. They thus sparked discussions about nuclear technology in space, which were further fueled when Cassini planned to leave Earth with a whopping 33 kg (72 lbs) of plutonium aboard. As a result, safety regulations got stronger, as did testing. “They wanted proof of the worst that could happen, so we did our best to smash them, blown them up, shoot them and break them”, said NASA test engineer Mary Ann Reimus.
Cassini launched despite its critics. Space-bound RTGs have an impressive safety record. For example, after the US satellite Nimbus-B had to be destroyed during launch in 1968, its plutonium pellets were recovered intact from the bottom of the sea off the coast of California to be re-used on a later mission.
The future
Nuclear power is an integral part of plans for future space missions, especially for outposts on the Moon and Mars. It remains unbeaten when it comes to energy density and reliability in dark, cold, and harsh conditions.
As power needs rise, we leave the realm of nuclear batteries. Previous missions used a couple of hundreds of watts, but a future mission to Mars is estimated to need orders of magnitude more. That — as well as a shortage of plutonium — is why plans for future outposts will likely rely on fission reactors. And innovation doesn’t stop there.
The story of nuclear power in space exploration thus seems to be only beginning. From its humble origins powering space probes and rovers, nuclear power is set to underpin mankind’s reach for other planets… and beyond.
Cassini launched despite its critics. Space-bound RTGs have an impressive safety record. For example, after the US satellite Nimbus-B had to be destroyed during launch in 1968, its plutonium pellets were recovered intact from the bottom of the sea off the coast of California to be re-used on a later mission.
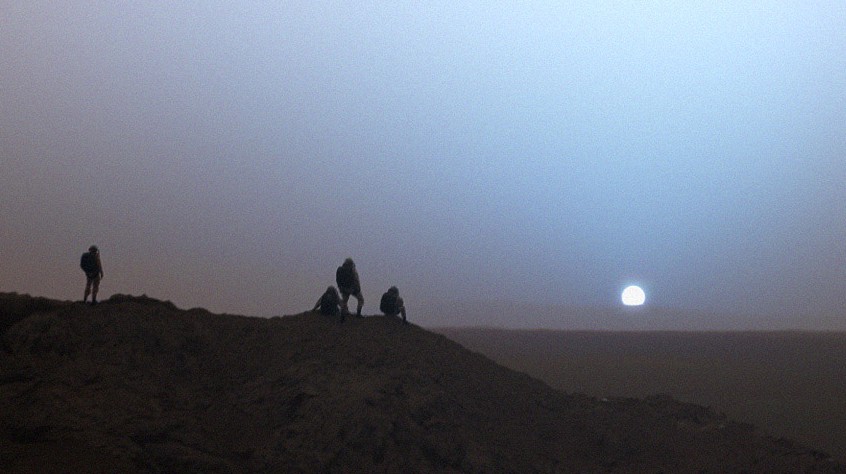